November 2, 2018, ©. Leeham News: In last week’s Corner we looked deeper into the fundamentals of the Mach 1.4 engine of Aerion’s AS2 SST, the GE Affinity.
Now we start looking at engines for a faster SST, up to the Mach 2.2 of the Boom Supersonic project.
To understand the fundamental problems of Mach 2 SST engines we will use the GE Affinity engine in a Mach 2 application and compare it to Concorde’s engine, the Rolls-Royce Olympus.
It will show where the fundamental differences are between an engine which can pass the Stage 5 noise regulations and work well at Mach 1.4 but which can’t be used for a Mach 2 application and an engine which works well at Mach 2 but can’t be used on aircraft taking off and landing at today’s civil airports.
Before we start, let’s repeat how we get thrust: Thrust = Mass Flow * Specific Thrust
If we take a Supersonic engine like Affinity and increase the cruise speed to Mach 2, we will increase the critical last stage compressor temperature (T3) by 50°C. If our design has the margins to handle it, we would have an engine delivering 4,700lbf of Net thrust while developing a Gross thrust of 113,000lbf at Mach 2 and 50,000ft.
The 5.3 times higher Gross thrust is needed to overcome the increased momentum drag of 108,300lbf, also called Ram drag. We have 150kg/s of air which enters the engine and which must be accelerated to Mach 1.5 before passing the fan. This mass flow then is ejected from the engine at a Specific Thrust (overspeed) of 140m/s.
The necessary high Gross thrust to generate the 4,700lbf of forward thrust sends the fuel consumption through the roof. We have a TSFC of 1.4lb/lbf/h, 55% higher than the same engine at Mach 1.4 and 17% higher than the Concorde’s engine.
The high fuel consumption is also because the engine has to work harder to compress the air before it enters the combustor. The air enters the engine at 150°C after the compression in the intake instead of 30°C for a Mach 1.4 application. This increases the work to increase the pressure in the compressors with 40%, an increase in power which is taken from the turbines.
In summary, we have a BPR 3 engine which has the core to give close to 30,000lbf static thrust at sea level. We run it at 18,000lbf at takeoff to get a Specific Thrust below 350m/s for noise reasons. Mass flow is then 225kg/s.
This engine gives us 4,700lbf of Net thrust at Mach 2 with a TSFC of 1.4 lb/lbf/h. Mass flow is 155kg/s, Specific Thrust 140m/s and with Gross thrust at 113,000lbf Ram drag is 108,300lbf. Gross to Net thrust ratio is 5.3.
The Concorde engine in comparison had a non-afterburner Static thrust of 31,000lbf with a Mach 2 thrust at 50,000ft of 11,700lbf. Its Static mass flow was 190kg/s with a Specific Thrust of 750m/s (the 900m/s was with afterburner). At Mach 2 the mass flow was 100kg/s and the Specific Thrust 400m/s.
The lower mass flow decreases Ram drag and gives less mass of high-temperature air to compress while developing more than double the Net thrust of the Affinity. The result is a TSFC of 1.2lb/lbf/h. The key to the higher cruise efficiency is the lower mass flow and higher Specific Thrust. The engine needs to produce 30,000lbf of Gross thrust to generate a forward thrust of 11,700lbf.
The design mantra for high-speed engines is low mass flow and high Specific Thrust. But it gives a high noise level at airport speeds or a low thrust.
If we throttle back the Concorde engine to a noise acceptable 350m/s Specific Thrust for takeoff, the engine produces 12,000lbf of thrust, 50% less than a throttled back Affinity.
The above shows us the contradiction between the design criteria for a high-speed SST engine and an environmentally acceptable engine. It also shows the contradiction between what we need for high-speed cruise and low-speed takeoff and landing.
How do we then get to an engine for a Mach 2 SST or a still more challenging Mach 2.2 SST (where the Affinity Gross to Net thrust ratio has grown to 7.7)?
This is the subject of next week’s Corner.
“The key to the higher cruise efficiency is the lower mass flow and higher Specific Thrust.”
So what’s going on with modern fighter jet engines such as on F15 and Tornado which are turbofans, though much lower bypass than 3:1 (and no quieter than Concorde was)? I take it the usual rules of propulsive efficiency still apply, moving a greater mass slower = better, but that’s in contradiction to the statement above.
Drawings of future civil turbofans sometimes involve an intercooler – you’d think such a thing would make its first appearance on a supersonic fighter engine, using fan air. Has there been such a device, and would cooling the gas in the compressor make any difference to the difference between net and gross thrusts?
Fighters don’t generally cruise at high Mach for thousands of miles. they sprint for short periods (either getting to the fight or getting away) and spend most of their time cruising around at roughly airliner speeds.
Even the supercruise capable F-22 rarely uses that capability and when it does it is only going about M1.4. additionally, the F119 engine is a roughly 1:1 BPR engine with relatively high specific thrust.
The Eurofighter Typhoon engine, EJ200, which has a BPR of 0.4 will be discussed next week. It’s typical of a military turbofan which has been designed to function well at both subsonic and high supersonic speeds.
Hi Chris,
“I take it the usual rules of propulsive efficiency still apply, moving a greater mass slower = better”.
Yes, it still applies but is overshadowed by the momentum drag problem. The Affinity with its low overspeed/Specific Thrust of 140m/s has a propulsive efficiency of 89% at M2. But it doesn’t help when the Ram drag is 108,000blf of a produced 113,000lbf Gross thrust.
It only shows how complex the total math around a Turbofan is. We have learned BPR and Propulsive efficiency is the “be all”. In effect it’s not, we also have momentum drag (and many other key parameters) but it’s not a dominant problem when airliners fly below M0.85. At M0.85 cruise speed, the air entering the engine only has to be accelerated by M0.35 before entering the engine, at M2.2 by M1.7.
Thus the designers for airliner turbofans can design for a lot of air accelerated to a modest overspeed, to gain propulsive efficiency. The Ram drag is still manageable. At M2.2 the designer has to design for low Ram drag and a low diameter engine as the key parameters. He designs a high Specific Thrust engine. Propulsive efficiency is then a secondary parameter.
Nice article, I would double check the “Gross thrust of 113,000lbf at Mach 2”.
Looking at the GE F-120 Engine and its successors they have a variable bypass ratio that should suit a SSBJ pretty well. https://www.geaviation.com/military/engines/ge-adaptive-cycle-engine
Hi Claes,
yes it’s 113klbf and 135klbf at M2.2.
M2 SSTs are the first aircraft who really needs a variable cycle engine. The military can go with a low bypass turbofan which works well at high speed and forget about the takeoff noise.
Didn’ t they do variable cycle on the y-12s j58 and all Problem they had was a lack of automated control to position the shock?
The J58 used in the A12 and SR71s were single spool straight jets with a very low compression ratio (to combat to high T3 at Mach 3). Like other engines, it had bleed ports between the low compressor section and the high compressor. These are opened to draw more air through the compressor ahead of the bleed port at certain load conditions (low RPM is one of these), otherwise, the angle of attack of the compressor blades can be too high and the compressor stalls. For the J58 one connected the bleed ports via tubes to the afterburner, by it supplying cooling air and increasing the Oxygen in the afterburner.
It also increased the mass flow slightly without increasing RPM and by it Specific Thrust, which increased propulsive efficiency when the engine employed bleed. You can call it the first (sometimes) leaky jet rather than a bypass engine as the bypass was not constant.
At speeds of Mach 3 you have so high compression in the inlet that the full compressor is not really needed, it only increases T3. It seems probable the engine control then opened the bleed to tap some air directly to the afterburner. It lowers the compressor work (which is hard work with 400°C air entering the engine at Mach 3), lowers compression (not a big deal with the multi-shock inlet doing the brunt of compression) and lowers T3, good for engine life. It also increases engine efficiency slightly and improves conditions in the afterburner.
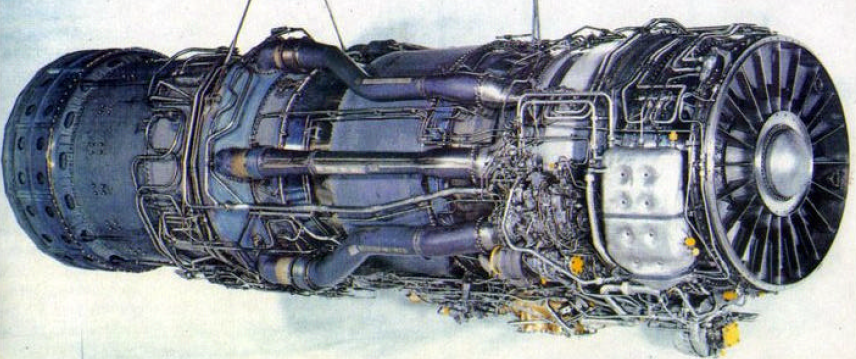
– – – – – Problem they had was a lack of automated control to position the shock? – – – – –
I may be wrong but I believe the SR-71 did have automated inlet cone control – just not digital computer-controlled automation. And I have seen several 1960s-era analog computers and pneumatic control systems on gas turbines replaced by “more sophisticated” sensors and digital controls systems that perform far worse than the analog ones they replace.
Yes. It was ‘electronic’ but now would be considered as analogue rather than digital.
For the Concorde, they used a specialised company Ultra Electronics Ltd, who built the first ‘airworthiness certified ‘ engine control for the Bristol Britannia, to provide electronic controls for :
engine control
variable geometry intake doors and afterburner doors
Ultra Electronics still exists and works in aviation field among others
This is a good overview of the J58 operation:
https://www.youtube.com/watch?v=F3ao5SCedIk
Hi Bjorn,
Excellent analysis. It is quite obvious that ram drag is a very important issue at very high vehicle speeds.
One correction: The air has to be “decelerated” to M 0.5 for the fan/compressor to handle it. That is why you need to decelerate from M2.2 to M0.5 for Concorde and M1.4 to M0.5 for Affinity.
Any idea what the component efficiencies (fan, compressor, turbine) are for Olympus and Affinity? The polytropic efficiencies must be significantly higher for Affinity. Can one assume that they are the same as CFM56 for the fan?
Just for the sake of completeness, I think you need to mention the L/D issue for SSTs, since that is what determines the drag and net thrust needed, and therefore the fuel consumption.
Hi Kant,
it’s accelerate as our reference frame is the ambient air. I’ve used this reference frame for the series instead of the Nacelle/Engine as it’s easier to understand the physics of momentum drag by observing an air molecule’s state before and after being hit by the SST intake.
The efficiency of the new designed low spool for the Affinity should be well above 0.9. For the CFM56 core, it should be unchanged unless GE redesigns the HP compressor and turbine, therefore around 0.9. Olympus had values just below 0.9.
As we talk TSFC we don’t need to involve the airframe’s drag and L/D. But L/D was low due to the volume and lift wave drag in addition to air friction and induced drag, around 7:1 dependent on where you are in the climbing cruise.
Bjorn,
Please permit me to make some back-of-the-envelope calculations for Concorde from data on Wikipedia (good enough for the purpose):
Cruise at 50000′ (~ 15 km) at M 2.02 means vehicle speed V = 596 m/s
Specific thrust ST= Ve – V = 400 m/s implies exhaust velocity Ve = 996 m/s. This also implies a propulsive efficiency = 2*V/(Ve+V) = 75%, quite good for a turbojet.
MTO mass of Concorde is stated to be 185,000 kg and MFL is 95,680 kg. Assuming a mass during cruise of say 150,000 kg and using stated L/D ratio of 7.14 at M 2.04, the drag is 206 kN or 46,300 lbf. This means that each engine must develop 11,600 lbf (~ 52 kN) during cruise, close to your 11,700 lbf value. This is the net thrust.
Gross thrust = mdot*Ve
Net thrust = mdot*(Ve-V)
Specific thrust = Ve-V
Using above values for Ve and V, we get Gross thrust ~ 29,000 lbf (~ 128 kN) and mass flow rate mdot ~ 129 kg/s.
Using the stated fuel consumption of 13.2 kg/km and cruise speed of 0.6 km/s, the fuel consumption is 7.92 kg/s. The total thrust from 4 engines is 206 kN and therefore the TSFC is 38.4 micrograms/second/N. Converting it to British units by dividing by 28.325, we get roughly 1.36 lbm/s/lbf. This is slightly higher than what Bjorn stated ~ 1.2 lbm/s/lbf. It all depends on what specific numbers you assume for L/D, cruise mass etc. and therefore some variance is to be expected.
Using 13.2 kg/km fuel burn and 0.8 fuel specific gravity and 120 PAX, we get a specific fuel burn (SFB) of 13.8 liters/PAX/100 km. Comparing this to SFB of say around 3.0 for modern aircraft, Concorde fuel burn is about 4.6 times.
The above numbers can be refined a bit with more accurate input parameters.
Following this but the tech details would take me a couple years to get into my head.
So I will follow with SFC in mind and leave the details to you guys!
I’m a bit confused at the math you’ve done here and would love some help!
You state that: “This engine gives us 4,700lbf of Net thrust at Mach 2 with a TSFC of 1.4 lb/lbf/h. Mass flow is 155kg/s, Specific Thrust 140m/s and with Gross thrust at 113,000lbf Ram drag is 108,300lbf. Gross to Net thrust ratio is 5.3.”
How is this a G/N thrust ratio of 5.3? It would seem to be 113000/4700 = 27.6.
Secondly, do you have any resources that we could use to recapitulate these calculations? For example, I don’t understand how you calculated the increase in the ram-drag from 7500 lbf at Mach 1.4 (Pt. 12 of this series) to 108,300lbf at Mach 2. Additionally, I’m confused at how you arrive at the TSFC numbers given.
Thanks so much!